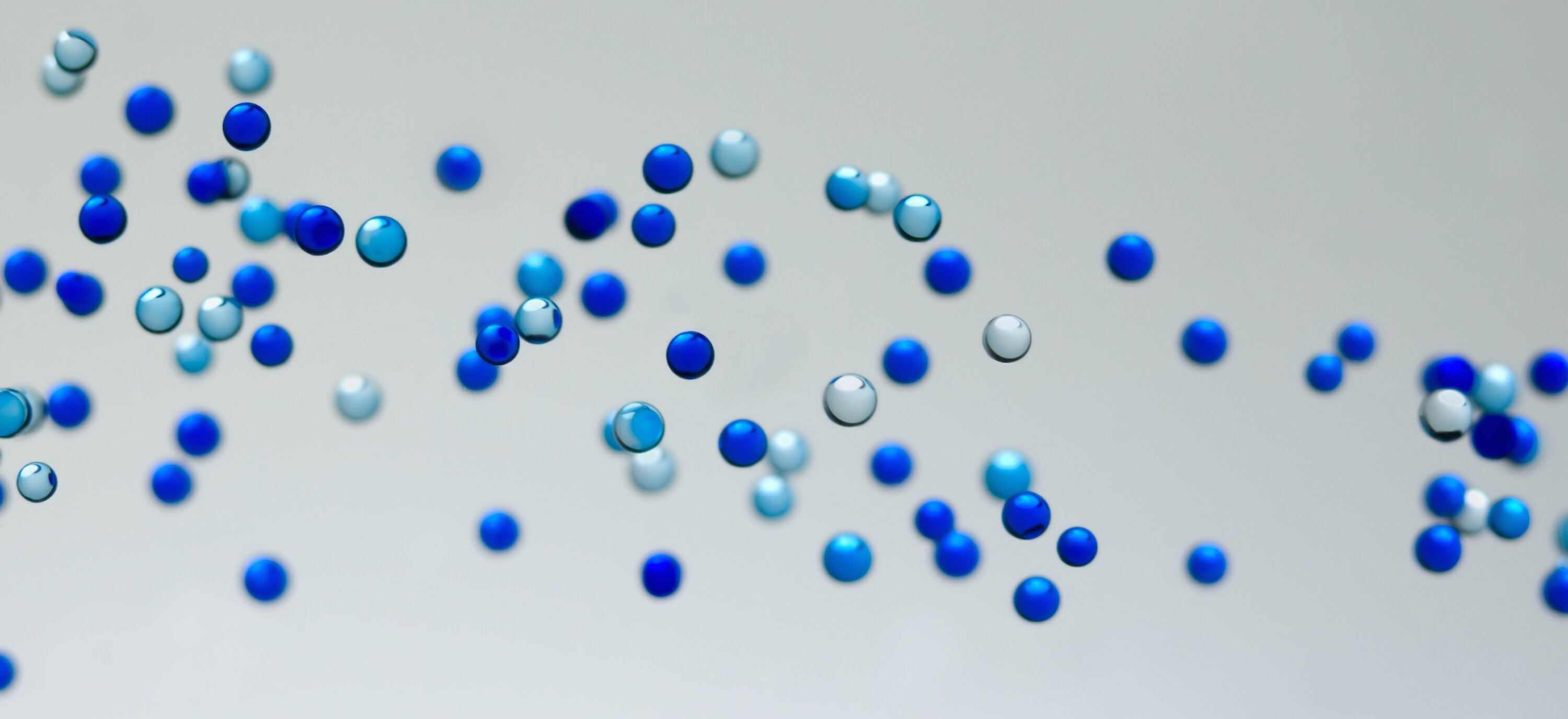
The capture of biological macromolecules and cells for molecular analysis of various bodily fluids like blood and fluids from clinical procedures like peritoneal washing and bronchoalveolar lavage aids in diagnostic testing and disease monitoring in patients. In the context of the multidisciplinary field of microfluidics – the intersection of engineering, physics, chemistry, microtechnology and biotechnology – biological molecules and cells are referred to as ‘particles’ because these are ‘objects’ that have physical or chemical properties like mass or volume. MGH investigators are manipulating complex microchannel geometries and fluid dynamics equations of motion to design new methodologies and microfluidic systems to separate bioparticles from their biological fluids and cellular suspensions to harness the rich molecular information contained within the body’s cells.
Read MoreInertial focusing and its complex parameters
Scientists in the pre-microfluidics 1960’s first reported experimental results on particles flowing through a straight channel in laminar flow (along streamlines), which showed that scattered flowing particles tended to form a thin, narrow ring known as an annulus between the centerline of the pipe and the pipe wall, and this discovery was called the ‘tubular pinch effect’. This newly observed behavior meant that the particles had to move across other streamlines to get to the center to form an annulus, a fluid movement previously thought to be impossible in a fluid flow lacking inertia (the tendency for objects to resist changes in their state of motion). Many investigators worked on follow-up studies to explain this perplexing motion of particles.
In fluid dynamics, the flow of fluid in a closed system can be described with two dimensionless Reynolds numbers that describe the unperturbed channel flow (channel Reynolds number) and parameters that describe both the particle and the channel (particle Reynolds number). Laminar flow occurs for low Reynolds number, with relatively slow velocity or high viscosity while turbulent flow is characterized by high Reynolds number. Laminar flow provides a smooth and predictable method for biomolecules and cells to be transported through microchannels. However, for the numerous instances where it is desirable to separate or concentrate these particles this laminar flow is a challenge as particles will always follow the streamlines which made the surprising tubular pinch effect difficult to explain.
After several decades of study, scientists finally determined that the tubular pinch behavior of particles in a laminar flow was caused by previously unknown forces on particles in an inertial flow – a combination of inertial lift forces acting on particles at higher particle Reynolds numbers. The migration across streamlines to equilibrium positions within the flow is due to inertial effects of the fluid motion around the particle and the interaction of this flow field with the walls of the channel. These forces are dependent upon the properties of the three contributing substances: (1) the particles, (2) the fluid in which the particles flow, and (3) the channel in which the particles within the fluid flow. Read more about the phenomenon of inertial focusing in Martel and Toner, 2014.
Applying inertial fluid dynamics in a microfluidic system

Schematic drawing of the inertial ordering process
Microfluidic technologies for particle and cell separation from bodily fluids first emerged about 20 years ago. One frustrating drawback with earlier microfluidics systems for particle separation had been their low operating flow rates. Small volumetric flow rates (which typically had ranged from 1-50 microliters per minute) made the precise collection of abundant cells possible but limited its utility for rare cells, such as circulating tumor cells, in large volumes of blood, where it would is necessary to process several milliliters within minutes.
MGH’s Dr. Mehmet Toner and his team of bioengineers and scientists in the early 2000’s became intrigued with concept of incorporating the effects of the inertia of the fluid in the system rather than limiting its effects as typically done in microfluidics. Reported in 2007 by then MGH postdoctoral fellow Dr. Dino Di Carlo (now Associate Professor of Bioengineering and Biomedical Engineering at UCLA-Berkeley), the MGH investigators began to explore complex channel geometries to design experiments that could influence the inertial effects of fluid to control the positions of bioparticles within such flows.
By utilizing inertial lift forces in laminar flow within microchannels the investigators were able to align and order microparticles continuously and at high flow rates that were initially randomly distributed. By introducing more complex microchannel designs including repetitive curvature, the MGH investigators were able to reduce focusing of the particles from an annulus for four points, to two points, then to a single stream of ordered particles precisely positioned laterally, within the transverse plane of the channel, and longitudinally along the direction of flow. This work utilizing a purely hydrodynamic (passive) approach demonstrated that inertial focusing could be applied in microchannels to differentially order particles of different sizes, continuously, at high rates, and without external forces.
Novel findings about inertial focusing behaviors in curved microchannels
This initial report describing the application of inertial focusing to control cells as they flowed through a microchannel represented just the tip of the iceberg for the MGH investigators. Since this publication, the research team has continued its pioneering work in asymmetrically curved channel geometry, the dynamics of varying the sizes and composition of particles and then observing their focusing behavior in curved channels, and the principles behind the observed behaviors. Several novel behaviors of particles have emerged from these ongoing studies of inertial focusing dynamics in curved channels.
Bioparticle density and size. The investigators tested many different bioparticles of varying densities and sizes as well as rigid versus deformable (blood cells or droplets that can change shape without rupturing) particles and found that particles can be focused under all test conditions. The investigators showed for the first time that particles focus to different parallel streamlines within the transverse plane of the channel depending on particle size. Interestingly, when studying the flow behavior of deformable red blood cells, the investigators discovered a fourth dimension of rotational alignment, a characteristic previously not attributed to the flow of red blood cells within a microchannel.

Brightfield and fluorescent images at select flow conditions grouped by channel width (horizontal) and flow rate (vertical)
Channel curvatures and velocities for quality focusing. In order to optimize devices for processing large volumes the researchers including Dr. Joseph Martel explored the effects of increasing the channel width and flow rate on the focusing behavior of microparticles in spiral microchannels. The investigators found that effective separation of focused particles can be achieved in low aspect ratio spiral microchannel designs (microchannel height to width of approximately 1/1 to 1/8) where the combined effect of lift and Dean drag forces can interplay to focus and order particle suspensions. They also discovered that focusing in microchannels breaks down into a primary and secondary fluorescent streak in the lowest aspect ratio channels at high average downstream velocities. The precursor to breakdown can be seen with streak movements away from the inner wall equilibrium position towards the center of the channel. This study concluded that as the channel became wider the best quality focusing occurred for a diminishingly smaller range of flow rates helping to understand the limitations of the phenomenon.
Uncoupling the Reynolds and Dean numbers. In a follow up on this previous work, Dr. Joseph Martel investigated the two main forces at work in curved channels by separating the effects of the non-dimensional parameters of Reynolds and Dean numbers, which traditionally were changed simultaneously at different flow conditions. By independently varying the Reynolds number, the investigators discovered two new complex behaviors of focusing that might allow the investigators to separate particles of an intermediate size and isolate a particle size of choice by varying the flow rate within a single microfluidic device rather than having a specific design for different applications.

Long-exposure fluorescence (LEF) and particle trajectory analysis (PTA) show particle migration towards a single centralized point along the channel centerline at high flow rates. Particle focusing in the HA solution persisted to Reynolds numbers well above the upper limit for particle focusing in water.
Inertio-elastic focusing of bioparticles. A previous study in the 1940s revealed that the addition of a polymer additive to a turbulent Newtonian fluid could reduce skin friction drag by up to 80%. This phenomenon has led to successful methods for reducing process costs for oil pipelines and increasing flow rate in firefighting equipment. Since then, additional studies have shown that fluid inertia and fluid elasticity can interact to stabilize a given flow if present simultaneously. To test whether such inertio-elastic effects can enable particle migration in microchannels at high Reynolds numbers, the MGH investigators (along with MIT investigators Thomas Ober and Gareth McKinley) used hyaluronic acid as a model viscoelastic drag-reducing additive and experimentally observed particle behavior at high flow rates. They showed that laminar microchannel flow not only can be achieved when both elasticity and inertia are present, but particle focusing can be accomplished at flow rates much higher than previously achieved in a microchannel. Read more about the phenomenon of inertio-elastic focusing in Lim and Toner, 2014.
Biomedical applications for inertial focusing in curved channels
Microfluidic technologies that rely on fluid inertia to drive lateral migration of bioparticles and cells to stable equilibrium positions at extremely high flow rates in microchannels are advancing science and medicine in unprecedented ways. These particle focusing techniques take advantage of microscale physics, with throughput comparable to macroscale systems, but without mechanical or electrical parts. The microfluidic systems are able to separate many kinds of living cells by differential inertial focusing – while maintaining cell viability and limiting cell damage. Today, blood components can now be easily separated for analysis, including platelets, which had proven to be challenging to separate from the white blood cells and red blood cells. Most recently, the MGH investigators have utilized inertial focusing in their new integrated microfluidic system called the “CTC i-Chip” to capture rare circulating tumor cells. Nanotechnology-based approaches like these allow scientists and clinicians to sort, capture, and manipulate single cells and single molecules for precise measurement of biomolecules and cells in vitro, manipulation of picoliter droplets, and clinical evaluation of complex samples.
Read more about the genesis of the CTC-iChip and the clinical testing results using the CTC-iChip.
Relevant publications
Lim EJ, Ober TJ, Edd JF, Desai SP, Neal D, Bong KW, Doyle PS, McKinley GH, Toner M. Inertio-elastic focusing of bioparticles in microchannels at high throughput. Nat Commun. 2014 Jun 18;5:4120. PubMed PMID: 24939508
Martel JM, Toner M. Inertial focusing in microfluidics. Annu Rev Biomed Eng. 2014 Jul 11;16:371-96. PubMed PMID: 24905880
Martel JM, Toner M. Particle focusing in curved microfluidic channels. Sci Reports. 2013 Nov 26;3:3340.
Martel JM, Toner M. Inertial focusing dynamics in spiral microchannels. Phys Fluids. 2012 Mar;24(3):32001. PubMed PMID: 22454556; PubMed Central PMCID: PMC3311666
Russom A, Gupta AK, Nagrath S, Di Carlo D, Edd JF, Toner M. Differential inertial focusing of particles in curved low-aspect-ratio microchannels. New J Phys. 2009 Jul 1;11:75025. PubMed PMID: 20862272; PubMed Central PMCID: PMC2942776
Di Carlo D, Edd JF, Irimia D, Tompkins RG, Toner M. Equilibrium separation and filtration of particles using differential inertial focusing. Anal Chem. 2008 Mar 15;80(6):2204-11. PubMed PMID: 18275222
Di Carlo D, Irimia D, Tompkins RG, Toner M. Continuous inertial focusing, ordering, and separation of particles in microchannels. Proc Natl Acad Sci U S A. 2007 Nov 27;104(48):18892-7. PubMed PMID: 18025477; PubMed Central PMCID: PMC2141878
Contact
Mehmet Toner, Ph.D. | Joseph Martel, Ph.D. | Eugene Lim, Ph.D. |
617-724-5336 | 617-724-5336 | 617-724-5336 |